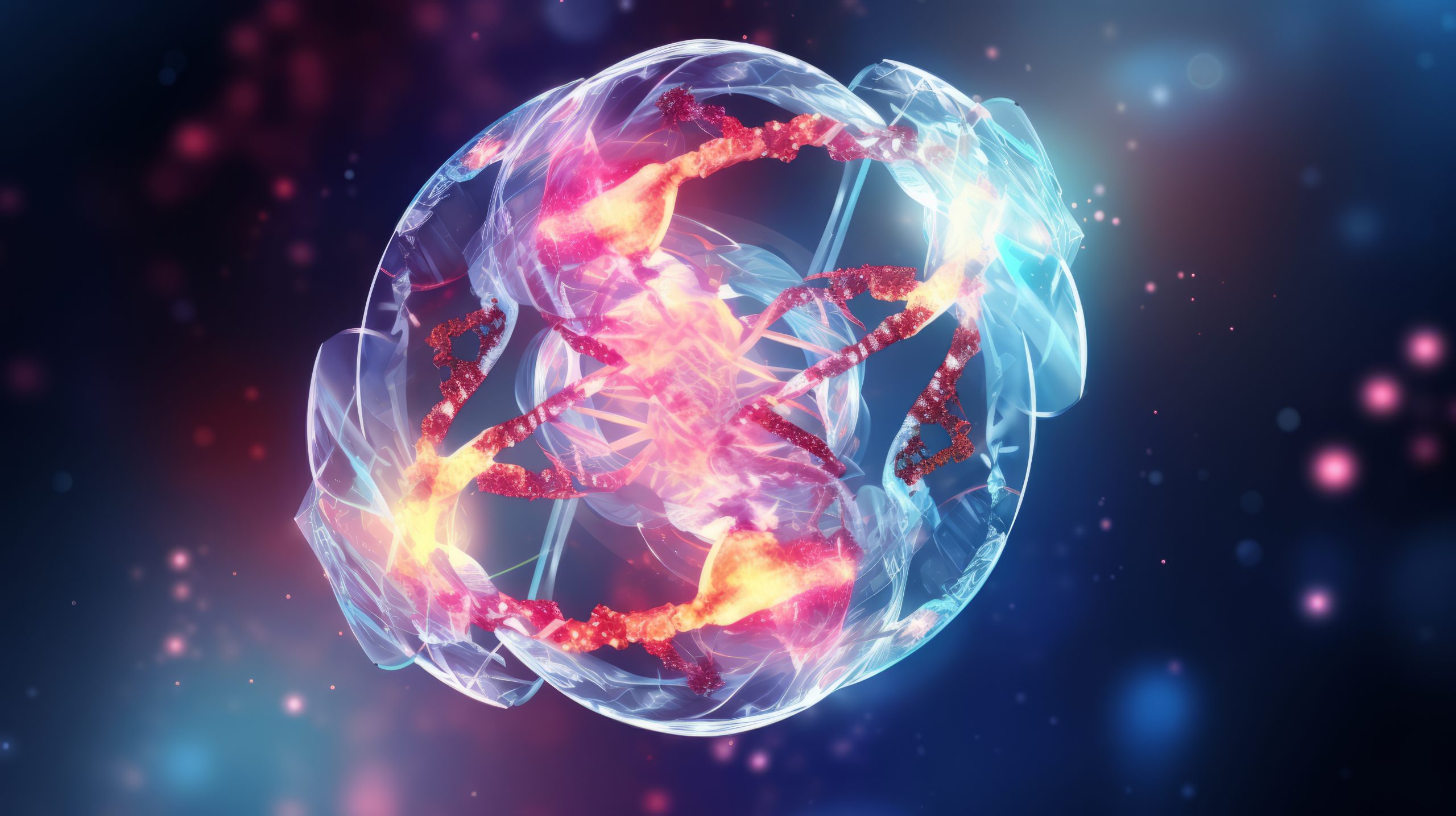
Deep inside the nucleus of every cell lies a long, slender molecule called DNA, the blueprint of life. If stretched out, the DNA in a single human cell would be about six feet long—an astonishing feat considering it’s packed inside a microscopic nucleus. But how does it fit, and more importantly, how does it function so efficiently despite being so tightly crammed? This is where the magic of DNA looping begins.
Imagine DNA as a giant book, filled with instructions written in the language of A’s, T’s, G’s, and C’s. But instead of reading the book linearly, the cell has a remarkable trick: it folds and loops the DNA, bringing distant chapters right next to each other. These loops are not random—they are carefully crafted by proteins, like tiny architects, ensuring that the right instructions are read at the right time.
The Loop-Makers: Proteins at Work
Meet the Lac repressor, one of the stars of this looping story. It’s a small protein, but it plays a big role. In bacteria, the Lac repressor acts like a gatekeeper for genes involved in digesting lactose (a sugar found in milk). When lactose isn’t around, the Lac repressor binds to the DNA and forms a loop, effectively locking away the lactose-digesting genes. But when lactose arrives, it changes the shape of the repressor, causing the loop to open and the genes to spring to life.
It’s like a well-timed performance, where the spotlight shines on a particular set of genes only when they’re needed.
The Physics of Loops: A Tug-of-War in the Nucleus
Now, imagine the forces at play. DNA looping is no gentle act—it’s a tug-of-war between the stiffness of the DNA and the binding force of proteins. DNA, like a coiled spring, resists bending. But proteins like the Lac repressor exert just the right amount of pressure to overcome this resistance, pulling the DNA into a loop.
It’s not just about brute force; it’s a dance of precision. The loop’s size, the distance between its ends, and the environment inside the cell all matter. Add macromolecular crowding—where other molecules jostle for space—and the looping game becomes even more intricate.
Why Loops Matter: Efficiency and Elegance
Why does DNA bother looping at all? The answer lies in efficiency. Looping allows genes to be regulated swiftly and precisely. It’s like creating shortcuts in a vast city—why take the long way when you can fold the map and bring two destinations together?
In humans, DNA looping is central to more complex processes. For instance, in immune cells, loops help rearrange DNA to create antibodies that fight off infections. In developing embryos, loops orchestrate the activation of genes at just the right moments, shaping everything from your heartbeat to your fingerprints.
A Labyrinth of Possibilities
Scientists are still unraveling the mysteries of DNA looping. How do these loops form? How are they maintained? And how do they interact with other cellular processes? Tethered particle motion microscopy, a fascinating tool, allows researchers to watch DNA loops in action. By tethering a DNA molecule to a tiny bead and observing its movement under a microscope, scientists can learn how proteins like the Lac repressor bind and bend DNA.
This isn’t just a tale of curiosity; it’s a story with profound implications. Understanding DNA looping could lead to breakthroughs in treating genetic diseases, engineering synthetic biological systems, or even designing better crops.
The Takeaway: Loops as Life’s Poetry
In many ways, DNA looping is a metaphor for life itself—full of twists, turns, and connections that make the whole greater than the sum of its parts. It’s a reminder that the beauty of biology often lies in its simplicity. A tiny loop of DNA, invisible to the naked eye, carries the power to shape organisms, influence evolution, and unlock the secrets of life.
The next time you think about your DNA, remember the loops. They’re not just a scientific phenomenon; they’re the poetry of your biology, weaving together the story of who you are.